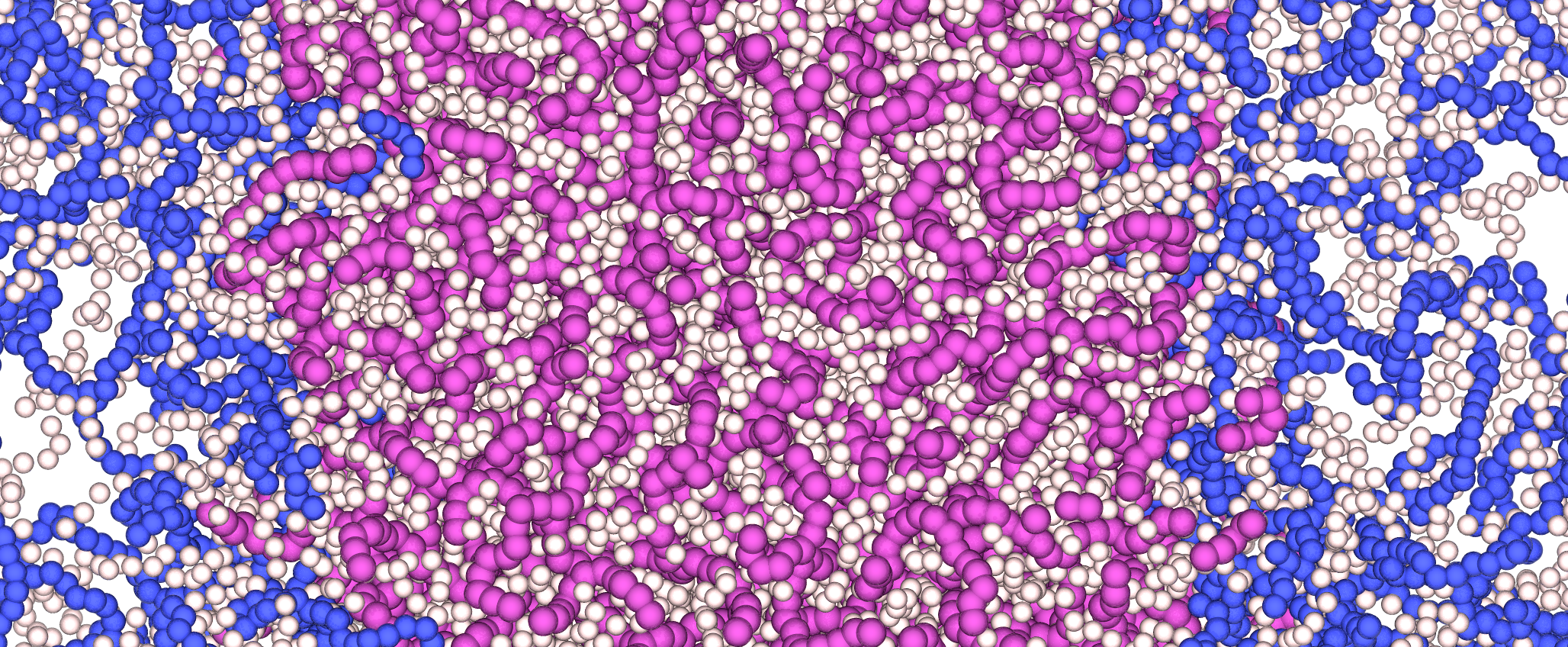
Jerelle Joseph co-authors study of a new model for understanding the driving forces that stabilise biomolecular condensates
The ability of Mpipi to describe interacting biomolecules at molecular resolution and physicochemical accuracy promises to provide paradigm-shifting views on the fundamental mechanisms that regulate the membraneless compartmentalisation of cells.
Jerelle Joseph et al
Our planet contains an outstandingly diverse population of species, from plants and animals, to microbes and viruses. Yet not all species are found everywhere, but rather, smaller subsets of them occupy different geographical regions, creating a wide-range of co-existing microenvironments that thrive under different climates and function in unique ways.
The same can be said of cells, which contain a huge number of different species, or biomolecules, that are carefully organised in space and time to fulfil a wide range of biological functions. Compartmentalisation allows cells to form diverse microenvironments with distinct conditions, concentrate and exclude specific molecules in different parts of the cell and achieve the coordinated control of thousands of simultaneous chemical reactions.
Although perhaps the most famous way in which cells achieve spatiotemporal organisation of biomolecules is the formation of membrane-bound organelles, the most widespread form of compartments completely lack membranes. In the place of membranes, these so-called biomolecular condensates are sustained and segregated in space by the physical chemistry of liquid–liquid phase separation (LLPS), analogous to oil drops dispersed in water.
While LLPS is suggested to have wide-ranging functional implications, its misregulation has been linked to the onset of neurodegenerative diseases and cancers. This has motivated major efforts to understand the biophysical principles that underlie biomolecular phase separation, and crucially, the sequence-dependent driving forces that stabilise phase-separated condensates. In particular, because LLPS is a collective phenomenon, molecular modelling and simulations have surfaced as powerful tools to understand the modulation of their phase behaviour.
Not surprisingly, over the past three years, many sequence-dependent coarse-grained models have been developed that compute phase diagrams of protein solutions with varying amino acid compositions. Their ultimate goal is to provide a quantitative description of the modulation of protein phase diagrams against specific amino-acid sequence changes and decipher the connection between protein sequence and phase behaviour.
In a new study published today in Nature Computational Science, Aleks Reinhardt, Rosana Collepardo-Guevara, Gates Cambridge Scholar Jerelle Joseph [2014] and colleagues describe a model they have developed to address this issue. Mpipi is a multiscale, sequence-dependent, coarse-grained description of proteins that has been carefully developed based on atomistic simulations, bioinformatics data and wealth of experimental information.
A key feature of the model is that it carefully balances out the contribution of each of the different amino acids to protein LLPS by extracting information from atomistic simulations of amino-acid pairs and bioinformatics data.
They compared Mpipi’s predictions with quantitative experimental phase diagrams of proteins in the temperature versus concentration space, with experimental radii of gyration of proteins and with seven other leading sequence-dependent coarse-grained models – which together represent the state-of-the-art in the field. Their findings show the effectiveness of Mpipi and that it can account for protein-RNA interactions and correctly predicts multiphase behaviour observed experimentally.
The researchers say: “The ability of Mpipi to describe interacting biomolecules at molecular resolution and physicochemical accuracy promises to provide paradigm-shifting views on the fundamental mechanisms that regulate the membraneless compartmentalisation of cells. Deciphering how biomolecular condensates behave at all scales, from molecules to whole condensates, is critical to advancing our understanding of their stability under both healthy and aberrant conditions, and holds great potential for uncovering mechanisms that underpin phase-separation-associated diseases.”
*Picture credit: Pin Yu Chew and Aleks Reinhardt